Top 12 Materials Scientist Skills to Put on Your Resume
In the competitive field of materials science, showcasing a set of specialized skills on your resume can significantly enhance your employability and career prospects. This article outlines the top 12 skills that materials scientists should highlight to stand out in the job market and excel in their roles.
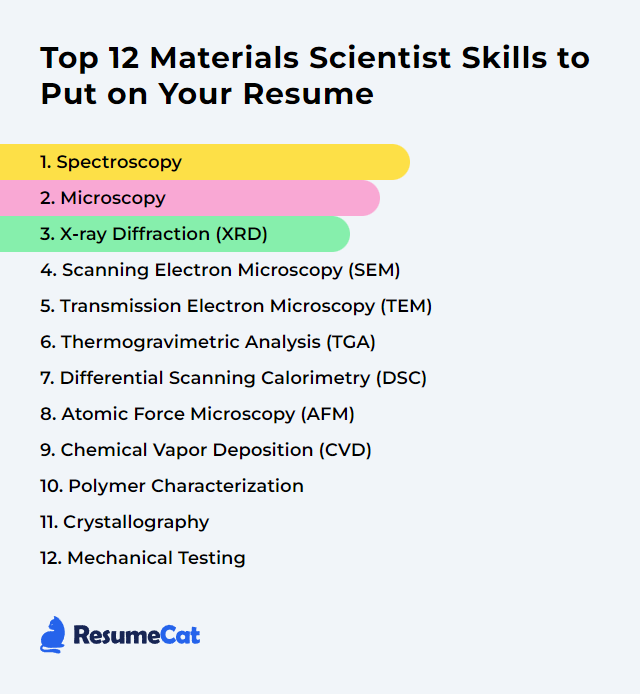
Materials Scientist Skills
- Spectroscopy
- Microscopy
- X-ray Diffraction (XRD)
- Scanning Electron Microscopy (SEM)
- Transmission Electron Microscopy (TEM)
- Thermogravimetric Analysis (TGA)
- Differential Scanning Calorimetry (DSC)
- Atomic Force Microscopy (AFM)
- Chemical Vapor Deposition (CVD)
- Polymer Characterization
- Crystallography
- Mechanical Testing
1. Spectroscopy
Spectroscopy is a technique used by materials scientists to analyze the composition and structure of materials by measuring their interaction with electromagnetic radiation across various wavelengths.
Why It's Important
Spectroscopy is essential for a Materials Scientist as it provides a powerful tool for characterizing materials, identifying their chemical composition, structure, and physical properties, enabling the development of new materials and the improvement of existing ones.
How to Improve Spectroscopy Skills
Improving spectroscopy techniques for a Materials Scientist involves enhancing resolution, sensitivity, and data analysis methods.
Instrument Calibration and Maintenance: Regular calibration and maintenance of spectroscopy equipment ensure accurate and reliable results. Photonics
Advanced Detectors and Light Sources: Incorporate advanced detectors and modern light sources to improve sensitivity and selectivity. Nature Reviews Methods Primers
Software and Data Analysis: Utilize sophisticated software for data acquisition and analysis. Implement machine learning algorithms to decipher complex spectra. Analytical Chemistry
Sample Preparation: Optimize sample preparation techniques to reduce noise and enhance signal quality. ScienceDirect
Multimodal Spectroscopy: Combine different spectroscopy techniques to gather complementary information for a more comprehensive analysis. ACS Publications
By focusing on these areas, Materials Scientists can significantly improve the performance and capabilities of spectroscopy in their research.
How to Display Spectroscopy Skills on Your Resume
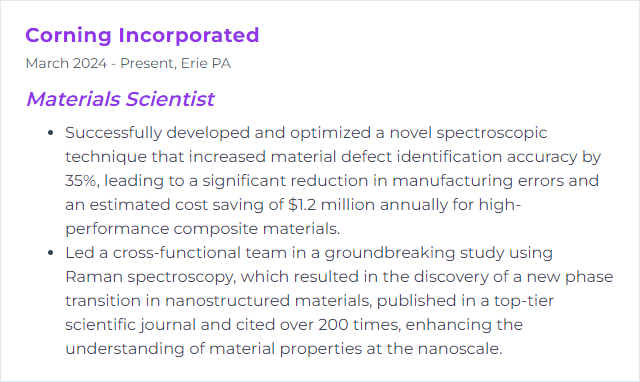
2. Microscopy
Microscopy, in the context of materials science, is a technique used to visualize and analyze the microstructure, composition, and properties of materials at microscopic levels, aiding in understanding their behavior and performance.
Why It's Important
Microscopy is crucial for a Materials Scientist as it enables the visualization, analysis, and manipulation of materials at the micro and nano scales, uncovering structural, compositional, and functional properties critical for developing new materials and improving existing ones.
How to Improve Microscopy Skills
Improving microscopy for a materials scientist involves enhancing resolution, contrast, and sample preparation techniques to better analyze materials at the micro or nanoscale. Here's a concise guide:
Enhance Resolution: Employ advanced techniques like Scanning Electron Microscopy (SEM) or Transmission Electron Microscopy (TEM) for higher resolution images. Consider super-resolution techniques or Atomic Force Microscopy (AFM) for surface topology studies.
Increase Contrast: Utilize staining methods or phase-contrast microscopy for better visualization of materials' microstructures. Techniques like Energy-dispersive X-ray spectroscopy (EDX) integrated with SEM can provide elemental composition insights.
Improve Sample Preparation: Optimize cutting, polishing, and thinning techniques for TEM or SEM analysis to maintain sample integrity. Explore Focused Ion Beam (FIB) milling for precise sample preparation.
Digital Image Processing: Use software for image enhancement and analysis, such as ImageJ or Gatan Microscopy Suite® Software for TEM images, to quantify and interpret microscopy data accurately.
Stay Updated with Technological Advances: Continuously explore emerging microscopy techniques and tools through academic journals and conferences in the field of materials science and microscopy.
By focusing on these areas, materials scientists can significantly improve the outcomes of their microscopy analyses, leading to deeper insights into material properties and behaviors.
How to Display Microscopy Skills on Your Resume
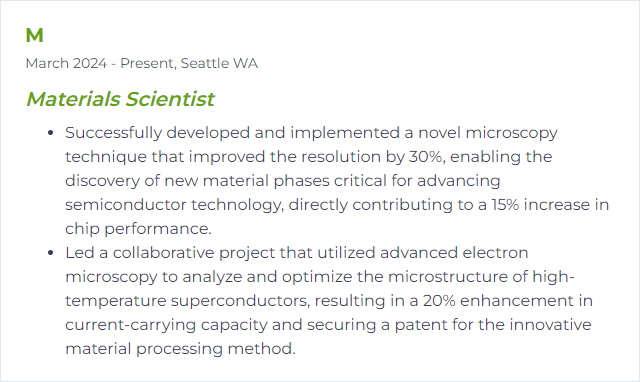
3. X-ray Diffraction (XRD)
X-ray Diffraction (XRD) is a powerful analytical technique used by materials scientists to determine the crystalline structure of a material. It involves directing X-rays at a sample and analyzing the diffraction pattern to identify lattice spacings and orientations, enabling the characterization of crystal phases, orientations, and defects.
Why It's Important
X-ray Diffraction (XRD) is crucial for Materials Scientists because it provides a direct method to determine the crystallographic structure, phase identification, and crystallinity of materials, enabling insights into material properties, behavior under different conditions, and guiding the development of new materials and improvement of existing ones.
How to Improve X-ray Diffraction (XRD) Skills
Improving X-ray Diffraction (XRD) analysis can significantly enhance materials characterization. Here are concise strategies for a Materials Scientist:
Sample Preparation:Ensure homogeneous and well-prepared samples to avoid texture and preferred orientation effects. Grinding and pressing the powder into a flat sample holder can improve the diffraction pattern's quality. International Union of Crystallography
Instrument Calibration:Regular calibration of the XRD instrument with standard samples (e.g., silicon) ensures accurate angle measurements. Rigaku Journal
Optimize Scanning Parameters:Adjust step size, scan speed, and range according to your sample's needs to balance between resolution and acquisition time. The choice of detector and X-ray wavelength (Cu, Mo, etc.) also plays a crucial role. Bruker Application Notes
Use of Software for Phase Identification and Quantification:Utilize advanced software for more accurate phase identification, quantification, and crystal structure analysis. PANalytical X'Pert HighScore Plus
Thin Film Analysis:For thin films, grazing incidence XRD (GIXRD) can be used to minimize substrate signals and enhance the film's diffraction. Journal of Applied Crystallography
Temperature and Pressure Studies:Performing XRD under varying temperatures and pressures can provide insights into phase transitions and stability. High-temperature XRD and diamond anvil cells are tools for such analyses. Review of Scientific Instruments
Data Analysis Techniques:Employ Rietveld refinement for better quantification of phase amounts and crystallographic information from the diffraction data. International Centre for Diffraction Data
Advanced Techniques:Explore advanced XRD techniques like synchrotron radiation for higher resolution and small-angle X-ray scattering (SAXS) for nanoparticle analysis. European Synchrotron Radiation Facility
Remember, the key to improving XRD analysis lies in meticulous sample preparation, precise instrument settings, and the adept use of advanced analysis techniques and software.
How to Display X-ray Diffraction (XRD) Skills on Your Resume
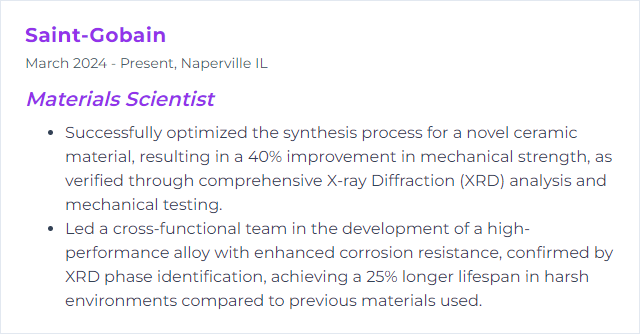
4. Scanning Electron Microscopy (SEM)
Scanning Electron Microscopy (SEM) is a powerful imaging technique used in materials science to obtain high-resolution images of materials' surfaces by scanning them with a focused beam of electrons. It reveals detailed surface features, composition, and morphology at the micro and nanoscale, essential for analyzing the structure-property relationships in materials.
Why It's Important
For a Materials Scientist, Scanning Electron Microscopy (SEM) is crucial as it enables the high-resolution imaging of material surfaces, revealing microstructural details, composition variations, and defects, which are essential for understanding material properties and guiding the development of new materials and improvements in existing ones.
How to Improve Scanning Electron Microscopy (SEM) Skills
Improving Scanning Electron Microscopy (SEM) for a Materials Scientist involves several key strategies aimed at enhancing image quality, resolution, and sample analysis capabilities:
Optimize Sample Preparation: Proper sample preparation is crucial. Ensure the sample is clean, properly mounted, and, if non-conductive, sputter-coated with a thin conductive layer (e.g., gold or carbon) to prevent charging. Microscopy Today provides insights and tips on sample preparation tailored for SEM.
Adjust Accelerating Voltage and Working Distance: Use the optimal accelerating voltage for your sample type. Lower voltages can improve surface detail observation, while higher voltages are useful for deeper penetration and compositional analysis. Adjusting the working distance can also help in optimizing resolution and depth of field. The National Center for Electron Microscopy offers resources on these adjustments.
Utilize Advanced Detectors: Employ secondary electron detectors for topographic information and backscattered electron detectors for compositional contrast. In-lens detectors can provide higher resolution images. For specific materials science applications, consider using energy-dispersive X-ray spectroscopy (EDS) detectors for elemental analysis. The Microscopy Society of America is a valuable resource for information on advanced detectors.
Implement Image Processing Techniques: Use software for image processing to enhance contrast, remove noise, and perform quantitative analysis. Open-source software like ImageJ can be particularly useful for image enhancement and analysis.
Continuous Learning and Training: Stay updated with the latest SEM techniques and technologies through workshops, webinars, and courses offered by professional societies and manufacturers. JEOL and Thermo Fisher Scientific provide educational resources and training opportunities.
By focusing on these areas, Materials Scientists can significantly improve the capabilities of SEM in their research and development projects.
How to Display Scanning Electron Microscopy (SEM) Skills on Your Resume
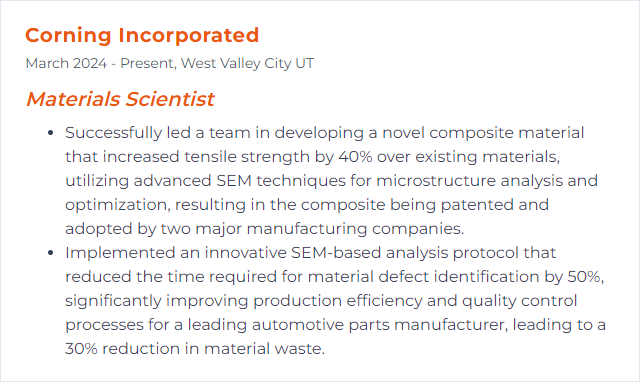
5. Transmission Electron Microscopy (TEM)
Transmission Electron Microscopy (TEM) is a microscopy technique in which a beam of electrons is transmitted through a very thin specimen to analyze its microstructure, chemical composition, and crystallographic information at the atomic or nanometer scale, crucial for materials science research and development.
Why It's Important
Transmission Electron Microscopy (TEM) is crucial for Materials Scientists because it enables the direct observation of the atomic and microstructural characteristics of materials, providing invaluable insights into their properties, behaviors, and performance at the nanoscale.
How to Improve Transmission Electron Microscopy (TEM) Skills
Improving Transmission Electron Microscopy (TEM) for materials science applications involves optimizing sample preparation, mastering imaging techniques, and employing advanced data analysis methods. Here's a concise guide:
Sample Preparation: Ensure ultrathin, defect-free samples for high-resolution imaging. Techniques like Focused Ion Beam (FIB) milling can create precise cross-sections of materials.
Optimize Imaging Conditions: Adjust the accelerating voltage and spherical aberration correction to enhance resolution and reduce beam-induced damage.
Advanced Detectors: Utilize state-of-the-art direct electron detectors for improved signal-to-noise ratio and dynamic range in imaging and diffraction.
Digital Micrography: Incorporate software tools for image processing and analysis to extract quantitative data from TEM images.
In-situ TEM: Apply in-situ TEM techniques to observe real-time changes in materials under various stimuli (e.g., heating, straining).
3D Reconstruction: Use electron tomography for three-dimensional reconstructions of materials at the nanoscale.
Continuing Education: Stay updated with the latest TEM developments and techniques through resources like the Microscopy Society of America and Journal of Materials Science.
Implementing these strategies will greatly enhance the capabilities of TEM for materials science research, facilitating groundbreaking insights into material structures and properties.
How to Display Transmission Electron Microscopy (TEM) Skills on Your Resume
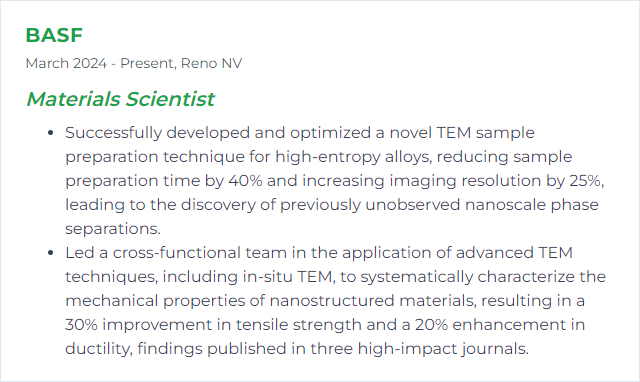
6. Thermogravimetric Analysis (TGA)
Thermogravimetric Analysis (TGA) is a technique used in materials science to measure the change in weight of a material as a function of temperature or time under a controlled atmosphere. This method helps in assessing thermal stability, decomposition temperatures, moisture content, and the composition of materials.
Why It's Important
Thermogravimetric Analysis (TGA) is crucial for Materials Scientists as it provides quantitative data on material decomposition, thermal stability, and compositional changes, essential for material design, selection, and improvement processes.
How to Improve Thermogravimetric Analysis (TGA) Skills
Improving Thermogravimetric Analysis (TGA) involves optimizing sample preparation, instrumentation settings, and data analysis to enhance accuracy, sensitivity, and repeatability. Here are concise strategies for a Materials Scientist:
Optimize Sample Size and Preparation: Ensure samples are of optimal size (typically a few milligrams) and homogeneously prepared to promote consistent thermal conductivity and reaction kinetics. Sample Preparation Techniques.
Calibrate and Validate Equipment Regularly: Regular calibration using standard materials ensures accuracy. Perform routine maintenance and validation to ensure the instrument's performance stability. Calibration and Maintenance.
Control Atmosphere: Use an appropriate purge gas (e.g., N2 for inert conditions, O2 for oxidative stability studies) at a suitable flow rate to control the reaction environment. Atmospheric Control in TGA.
Optimize Heating Rate: A slower heating rate can improve resolution by allowing more time for reactions to occur but may increase analysis time. Balance resolution with practical considerations. Effect of Heating Rate.
Employ Advanced Data Analysis Techniques: Utilize sophisticated data analysis software for deconvolution of overlapping decomposition steps and kinetic analysis. Advanced methods can also help in distinguishing between closely related materials or phases. Advanced Data Analysis.
Use Baseline Correction and Drift Compensation: Implement baseline correction to adjust for any instrumental drift or noise, ensuring more accurate weight loss measurements. Baseline Correction Methods.
Cross-Validate with Complementary Techniques: Enhance TGA results by combining them with data from complementary techniques like Differential Scanning Calorimetry (DSC), Fourier Transform Infrared Spectroscopy (FTIR), or X-Ray Diffraction (XRD) for a more comprehensive analysis. Complementary Techniques.
Implementing these strategies will significantly improve the reliability and insight gained from Thermogravimetric Analysis, thereby aiding in the development and characterization of materials with precision.
How to Display Thermogravimetric Analysis (TGA) Skills on Your Resume
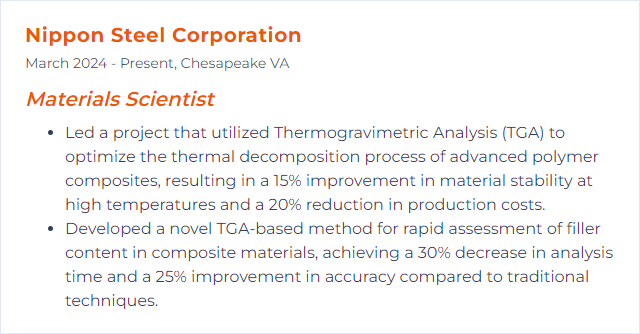
7. Differential Scanning Calorimetry (DSC)
Differential Scanning Calorimetry (DSC) is a thermal analysis technique used by materials scientists to measure the heat flows associated with thermal transitions in materials, such as melting, crystallization, and glass transitions, thereby providing insights into the material's thermal stability, composition, and purity.
Why It's Important
Differential Scanning Calorimetry (DSC) is crucial for Materials Scientists as it precisely measures material phase transitions, thermal stability, and heat capacities. This data is essential for characterizing materials, optimizing processing conditions, and predicting performance in various applications.
How to Improve Differential Scanning Calorimetry (DSC) Skills
Improving Differential Scanning Calorimetry (DSC) performance and accuracy involves several strategies tailored for materials scientists:
Calibration: Regularly calibrate the DSC using standards (e.g., indium, zinc) to ensure temperature accuracy and heat flow. NIST provides guidelines and standards for calibration.
Sample Preparation: Ensure uniform sample size, mass, and packing to reduce variability. Careful preparation can minimize thermal gradients and improve baseline stability. Elsevier’s guide on sample preparation offers practical tips.
Purge Gas: Use high-purity purge gases (nitrogen or argon) to minimize oxidation or other reactions that could affect the measurement. The flow rate should be optimized for your specific analysis. The TA Instruments website provides insights into the importance of purge gases.
Baseline Correction: Perform baseline corrections to account for instrumental drift and ensure accurate enthalpy measurements. This process can be software-assisted, as outlined in various DSC software manuals.
Scanning Rate: Adjust the heating/cooling rate to balance between resolution and sensitivity. Slower rates can improve resolution but may increase noise. PerkinElmer offers guidance on optimizing scanning rates.
Software Analysis: Utilize advanced software for data analysis to deconvolute complex transitions and compare experimental data with theoretical models. Software like TA Universal Analysis can enhance data interpretation.
Instrument Maintenance: Regular maintenance of the DSC instrument, including the furnace, sensors, and cooling systems, is crucial for consistent performance. Manufacturer websites often have maintenance tips and schedules.
Training and Experience: Continuous learning and practice are essential. Attend workshops, webinars, and courses offered by DSC manufacturers and academic institutions.
Improving DSC analysis is a continuous process of calibration, careful sample preparation, and methodical experimentation, supported by up-to-date training and maintenance.
How to Display Differential Scanning Calorimetry (DSC) Skills on Your Resume
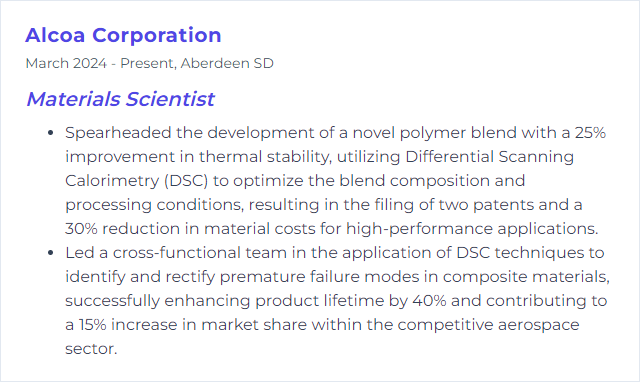
8. Atomic Force Microscopy (AFM)
Atomic Force Microscopy (AFM) is a high-resolution imaging technique used by materials scientists to visualize, measure, and manipulate materials at the nanoscale. It operates by scanning a sharp tip attached to a cantilever over a sample surface to map out the topography, mechanical properties, and other surface characteristics at atomic to micron scales.
Why It's Important
Atomic Force Microscopy (AFM) is crucial for Materials Scientists because it enables the nanoscale characterization of surfaces, providing insights into material properties, structure, and behavior at an atomic level, essential for materials design and innovation.
How to Improve Atomic Force Microscopy (AFM) Skills
To improve Atomic Force Microscopy (AFM) for applications in materials science, consider the following strategies:
Enhanced Probes: Use specialized probes such as high-aspect-ratio or super-sharp tips to achieve higher resolution and minimize tip-sample convolution effects. Bruker offers a variety of advanced AFM probes.
Advanced Imaging Modes: Implement advanced imaging modes like PeakForce Tapping® and TappingMode™ for better surface characterization and reduced tip-sample forces, which minimizes sample damage. Nanosurf provides insights into various imaging modes.
Environmental Control: Use AFM systems with environmental controls to study materials in their native conditions (e.g., under specific humidity or in liquid). This approach is crucial for studying biological materials or hydration effects. Park Systems offers solutions for in-liquid measurements.
Quantitative Measurements: Employ Force Spectroscopy and quantitative nano-mechanical mapping techniques to extract material properties (elasticity, adhesion, etc.) at the nanoscale. Asylum Research - An Oxford Instruments Company has resources on quantitative AFM techniques.
Integration with Other Techniques: Combine AFM with other analytical techniques such as Raman spectroscopy or fluorescence microscopy for correlated multiparametric analysis. This integration can provide comprehensive material characterization. WITec specializes in integrated AFM-Raman systems.
Software and Data Analysis: Utilize advanced software for image processing and data analysis to extract more information from your AFM data. Open-source software like Gwyddion offers a wide range of tools for AFM data analysis.
Training and Best Practices: Continuous learning about AFM operation, tip selection, and sample preparation can significantly improve results. Online resources and workshops provided by AFM manufacturers and academic institutions are invaluable.
By focusing on these areas, materials scientists can enhance the capabilities of AFM for detailed materials characterization, leading to new insights and advancements in materials science.
How to Display Atomic Force Microscopy (AFM) Skills on Your Resume
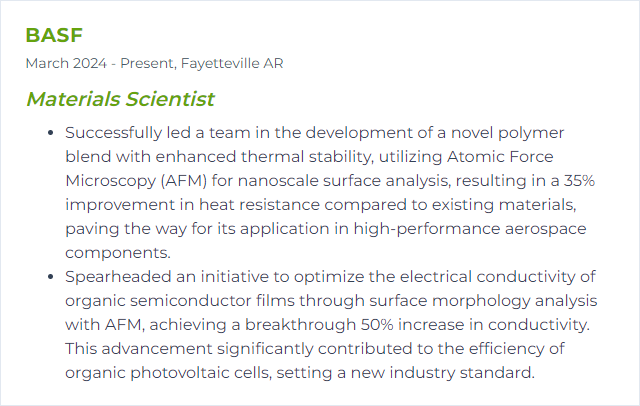
9. Chemical Vapor Deposition (CVD)
Chemical Vapor Deposition (CVD) is a materials engineering process where high-purity, solid materials are deposited on a substrate through the chemical reaction of vapor-phase precursors. This technique allows for the controlled growth of films, coatings, or even freestanding structures of various materials, including metals, semiconductors, and polymers, under specific conditions of temperature and pressure.
Why It's Important
Chemical Vapor Deposition (CVD) is crucial for a Materials Scientist because it allows for the controlled deposition of high-purity, high-performance solid materials with precise thickness and compositional control, enabling the fabrication of advanced materials and coatings for a wide range of applications, including electronics, optics, and wear-resistant surfaces.
How to Improve Chemical Vapor Deposition (CVD) Skills
Improving Chemical Vapor Deposition (CVD) processes involves optimizing parameters to enhance coating quality, efficiency, and material properties. Key strategies include:
Precursor Selection: Choose precursors with high purity and appropriate decomposition temperatures for targeted materials. Precursor Selection for CVD.
Temperature Control: Precisely control the substrate and reaction chamber temperatures to ensure uniform film growth and desired phase compositions. Temperature Effects in CVD.
Pressure and Flow Rates: Adjust the reactor pressure and gas flow rates to optimize the deposition rate and film uniformity. Gas Flow Dynamics in CVD.
Substrate Preparation: Proper substrate cleaning and surface preparation can significantly improve film adhesion and quality. Substrate Surface and CVD.
In-situ Monitoring and Control: Utilize in-situ diagnostic tools (e.g., Raman spectroscopy, optical emission spectroscopy) for real-time process monitoring and adjustments. In-situ Diagnostics for CVD.
By focusing on these aspects, materials scientists can tailor CVD processes to achieve desired material properties and coating characteristics more efficiently and with higher quality.
How to Display Chemical Vapor Deposition (CVD) Skills on Your Resume
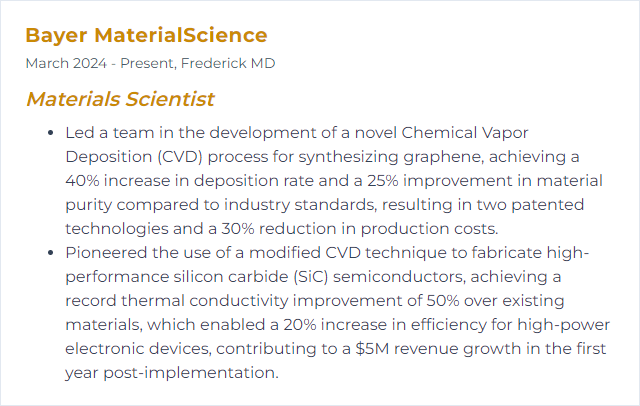
10. Polymer Characterization
Polymer characterization involves the analysis and understanding of a polymer's physical, chemical, and mechanical properties. It helps in determining the polymer's structure, molecular weight, composition, and thermal behavior, essential for tailoring materials for specific applications. Techniques include spectroscopy, chromatography, microscopy, and rheology, among others.
Why It's Important
Polymer characterization is crucial for a Materials Scientist because it provides essential information on polymer structure, composition, and properties, enabling the design and optimization of materials for specific applications, ensuring quality control, and guiding the development of innovative polymer-based solutions.
How to Improve Polymer Characterization Skills
Improving polymer characterization involves enhancing the accuracy, resolution, and information depth of techniques used to analyze polymers. Here are concise strategies tailored for a Materials Scientist:
Integrate Advanced Spectroscopic Methods: Incorporate advanced spectroscopic methods like Fourier Transform Infrared Spectroscopy (FTIR) and Nuclear Magnetic Resonance (NMR) to achieve detailed molecular structure characterization.
Utilize High-Resolution Chromatography: Employ Size Exclusion Chromatography (SEC) and High-Performance Liquid Chromatography (HPLC) for precise molecular weight distribution and compositional analysis.
Leverage Thermal Analysis Techniques: Refine thermal characterization by using Differential Scanning Calorimetry (DSC) and Thermogravimetric Analysis (TGA) to assess thermal transitions and stability.
Employ Advanced Microscopy: Implement Scanning Electron Microscopy (SEM) and Transmission Electron Microscopy (TEM) for high-resolution morphological and structural analysis.
Adopt Rheological Assessment: Use rheology for understanding the viscoelastic behavior of polymers by applying techniques like Rotational Rheometry and Oscillatory Rheometry.
Incorporate Multi-Technique Approaches: Combine multiple characterization techniques to obtain a comprehensive understanding of polymer properties. This multidimensional approach can be facilitated by resources like ScienceDirect for accessing relevant research articles and methodologies.
Stay Updated with Latest Developments: Continuously update your knowledge and skills by following leading journals like Polymer and Macromolecules, and attending workshops and conferences in the field of polymer science.
By integrating these advanced and multi-faceted strategies, materials scientists can significantly enhance the characterization of polymers, leading to deeper insights and innovation in materials design and application.
How to Display Polymer Characterization Skills on Your Resume
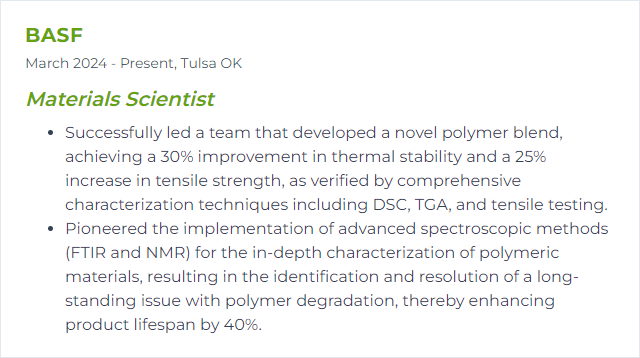
11. Crystallography
Crystallography is the study of crystal structures and their properties, enabling materials scientists to understand the arrangement of atoms in solids and how this influences material behavior and characteristics.
Why It's Important
Crystallography is crucial for materials scientists because it provides detailed information on the arrangement of atoms in solids, enabling the understanding of material properties, guiding the design of new materials, and optimizing existing ones for specific applications.
How to Improve Crystallography Skills
Improving crystallography skills, particularly for a materials scientist, involves both theoretical understanding and practical application. Here are concise tips:
Strengthen Theoretical Knowledge: Enhance your understanding of crystal structures, symmetry, and diffraction techniques. MIT's OpenCourseWare offers a comprehensive course on Crystal Structure Analysis.
Practical Experience with X-ray Diffraction (XRD): Gain hands-on experience with XRD equipment. The International Union of Crystallography provides valuable resources and guidelines, especially the Commission on Powder Diffraction.
Software Proficiency: Improve your skills in crystallography software for data analysis and modeling. Software like CRYSTAL MAKER and VESTA are essential tools.
Join Professional Communities: Engage with communities and forums like the American Crystallographic Association for networking, resources, and conferences.
Continuous Learning: Stay updated with the latest research and methodologies through journals and publications from sources like the Journal of Applied Crystallography.
By focusing on these areas, materials scientists can significantly improve their crystallography skills, leading to better analysis and understanding of materials.
How to Display Crystallography Skills on Your Resume
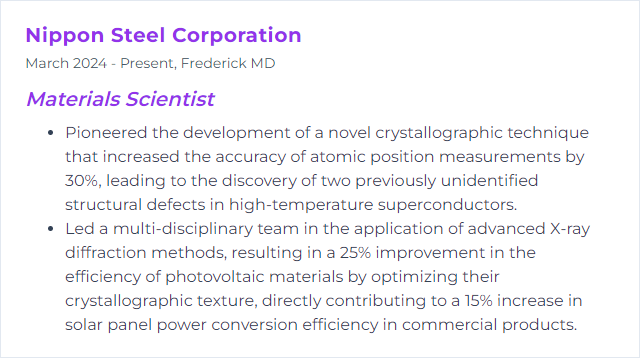
12. Mechanical Testing
Mechanical testing is the process used by materials scientists to evaluate the mechanical properties, such as strength, ductility, hardness, and toughness, of a material under various conditions of load, temperature, and strain. This helps in understanding the material's behavior and suitability for specific applications.
Why It's Important
Mechanical testing is crucial for a Materials Scientist as it provides essential data on the strength, elasticity, and ductility of materials, enabling the prediction of their behavior under different forces and conditions, which is vital for material selection, design, and ensuring safety and performance in real-world applications.
How to Improve Mechanical Testing Skills
Improving mechanical testing, especially from a materials scientist's perspective, involves enhancing accuracy, reproducibility, and relevancy of the tests to real-world applications. Here's a concise guide:
Standardization: Follow international standards (e.g., ASTM, ISO) to ensure consistency and comparability of results. ASTM International and ISO provide detailed protocols for various materials and test types.
Calibration and Maintenance: Regularly calibrate testing machines and equipment to avoid drifts in measurements. NIST offers guidelines on calibration practices.
Sample Preparation: Ensure consistent and precise preparation of specimens to minimize variability. Techniques should adhere to standards for dimensions and surface finish.
Environmental Control: Conduct tests under controlled environmental conditions (temperature, humidity) to reduce their impact on material properties. Information on environmental effects can be found through Materials Project which provides data on material properties.
Advanced Instrumentation: Use state-of-the-art testing equipment with higher precision and capabilities, including digital image correlation (DIC) for strain measurements and high-resolution sensors. Instron and MTS Systems are leaders in providing advanced mechanical testing equipment.
Data Analysis and Reporting: Utilize modern data analysis software for more accurate interpretation of test results. Ensure comprehensive reporting, including test conditions, equipment used, and analysis techniques. Software like MATLAB can be instrumental.
Training and Competence: Ensure personnel are well-trained and competent in test procedures, equipment operation, and data interpretation to reduce human error.
Collaboration and Benchmarking: Engage with research institutions and industry partners for knowledge exchange and benchmarking studies. This can lead to the adoption of best practices and innovative testing methodologies.
By implementing these strategies, materials scientists can significantly improve the reliability and utility of mechanical testing outcomes, leading to better material design and application.
How to Display Mechanical Testing Skills on Your Resume
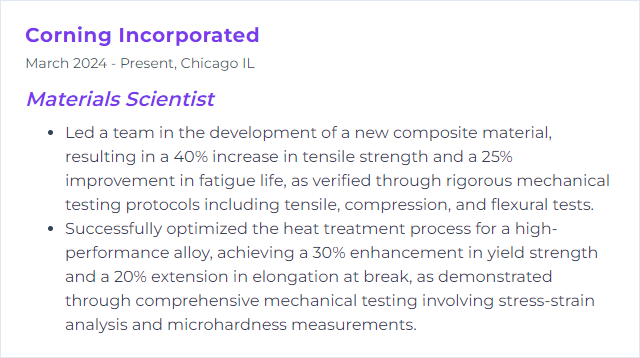