Top 12 Microwave Engineer Skills to Put on Your Resume
In the fast-evolving field of microwave engineering, showcasing the right blend of technical skills and practical expertise on your resume can set you apart in the competitive job market. Highlighting a comprehensive set of microwave engineer skills is crucial to demonstrate your ability to design, analyze, and improve complex microwave systems and components.
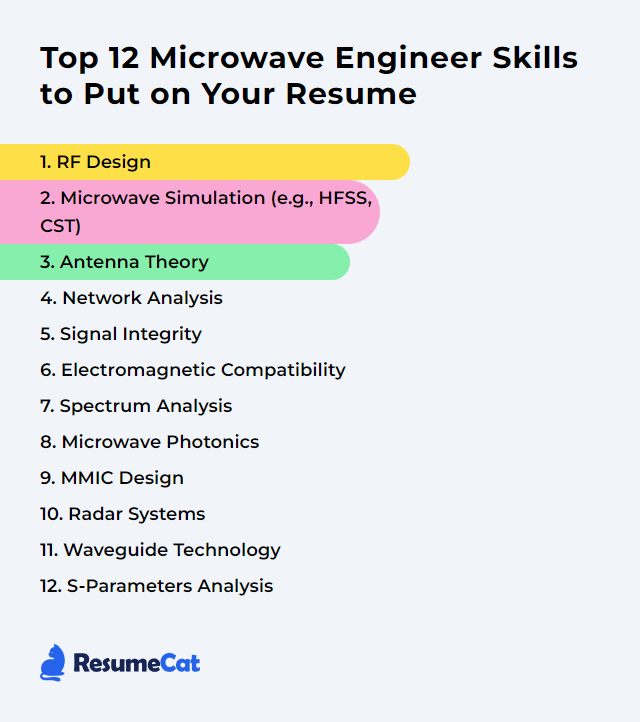
Microwave Engineer Skills
- RF Design
- Microwave Simulation (e.g., HFSS, CST)
- Antenna Theory
- Network Analysis
- Signal Integrity
- Electromagnetic Compatibility
- Spectrum Analysis
- Microwave Photonics
- MMIC Design
- Radar Systems
- Waveguide Technology
- S-Parameters Analysis
1. RF Design
RF Design, in the context of Microwave Engineering, involves the planning and crafting of systems and components (such as antennas, receivers, transmitters) that operate at radio frequencies, typically ranging from 3 kHz to 300 GHz, to achieve efficient transmission and reception of radio waves for various applications like communication, radar, and navigation.
Why It's Important
RF (Radio Frequency) design is crucial for a Microwave Engineer because it involves the development and optimization of circuits, systems, and devices that operate at microwave frequencies, enabling efficient transmission and reception of signals for telecommunications, radar, and various wireless applications.
How to Improve RF Design Skills
Improving RF (Radio Frequency) design, particularly for a Microwave Engineer, involves a few key strategies focused on enhancing performance, reducing interference, and ensuring efficiency. Here's a very short and concise description:
Simulation and Modeling: Use advanced simulation tools like ANSYS HFSS to accurately model and predict RF behavior before physical prototyping. This step is crucial for understanding the electromagnetic performance of RF components.
Component Selection: Choose high-quality components that match your design requirements. Pay attention to specifications such as frequency range, power handling, and noise figure.
Impedance Matching: Ensure optimal power transfer and minimize reflections by using impedance matching techniques. Tools like Smith Chart are invaluable for visualizing and solving matching problems.
Minimize Losses: Use low-loss materials and design strategies to reduce insertion loss. This includes selecting proper PCB substrates and minimizing the length of RF transmission lines.
Isolation and Shielding: Implement isolation techniques and shielding to protect sensitive components from external noise and to prevent leakage of the RF signal.
Layout Optimization: Optimize your PCB layout by carefully placing components to minimize cross-talk and using ground planes effectively to reduce noise. Cadence Allegro PCB Designer offers features tailored for RF design needs.
Thermal Management: Design for effective heat dissipation using heatsinks, thermal vias, and considering the thermal properties of your materials to ensure stable operation of RF components.
Testing and Iteration: Use spectrum analyzers and network analyzers to test your design extensively and iterate based on real-world performance. Keysight offers a range of tools for RF testing and measurement.
Stay Updated: Continuously update your knowledge with the latest advancements in RF technology and design practices by following IEEE publications and participating in forums like RF Globalnet.
By focusing on these areas, you can significantly improve the performance and reliability of your RF designs.
How to Display RF Design Skills on Your Resume
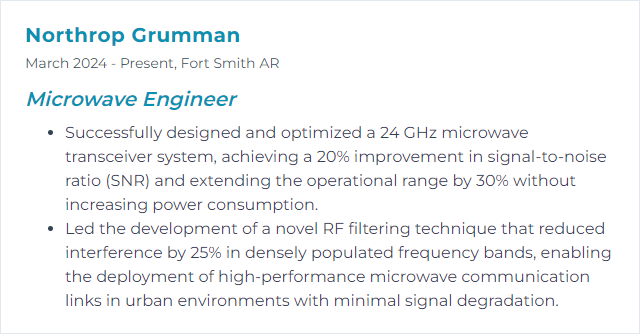
2. Microwave Simulation (e.g., HFSS, CST)
Microwave Simulation, using tools like HFSS (High-Frequency Structure Simulator) and CST (Computer Simulation Technology), involves the computational modeling and analysis of electromagnetic fields and waves in microwave frequencies. These simulations help microwave engineers design, optimize, and predict the performance of RF (Radio Frequency) components, antennas, and circuits before physical prototyping, ensuring efficiency and accuracy in developing microwave systems and devices.
Why It's Important
Microwave simulation tools like HFSS and CST are essential for microwave engineers as they enable accurate modeling of electromagnetic fields and interactions in microwave components and systems. This allows for the optimization of design parameters, prediction of performance, and reduction of costly prototyping and testing phases, ultimately speeding up the development process and enhancing the performance and reliability of microwave devices and systems.
How to Improve Microwave Simulation (e.g., HFSS, CST) Skills
Improving microwave simulation in software like HFSS (Ansys High-Frequency Structure Simulator) or CST (Computer Simulation Technology) involves a combination of refining the simulation setup, optimizing computational resources, and applying best practices in modeling. Below are concise strategies:
Mesh Refinement: Ensure the mesh is adequately refined in areas of high field intensity or where geometry details are small relative to the wavelength. Use adaptive meshing features when available. Ansys Meshing Guide.
Material Properties: Accurately input material properties. For novel or composite materials, measure dielectric properties at the operating frequency if possible. CST Material Modeling.
Boundary Conditions: Use appropriate boundary conditions (e.g., perfect electric conductor (PEC), perfect magnetic conductor (PMC), or radiation boundaries) to accurately model the environment without unnecessarily increasing computational load. HFSS Boundary Conditions.
Solver Selection: Choose the right solver for your problem type (e.g., frequency domain, time domain). Consider hybrid approaches for complex models. CST Solver Technology.
Parameter Sweep and Optimization: Use parameter sweeps to understand the effect of design variations. Apply optimization tools available within the software to fine-tune design parameters for performance. Ansys HFSS Optimetrics.
High-Performance Computing (HPC): Utilize HPC options for large or complex simulations to reduce computation time. This includes parallel computing and cloud resources. Ansys Cloud.
Post-Processing and Result Verification: Carefully analyze simulation results. Compare with analytical results or measurements from prototypes to verify accuracy. CST Result Visualization.
Continuous Learning: Stay updated with software updates, new features, and best practices. Participate in webinars, forums, and training sessions offered by software providers.
By methodically applying these strategies, microwave engineers can enhance the accuracy and efficiency of their simulations in HFSS, CST, or similar electromagnetic simulation tools.
How to Display Microwave Simulation (e.g., HFSS, CST) Skills on Your Resume
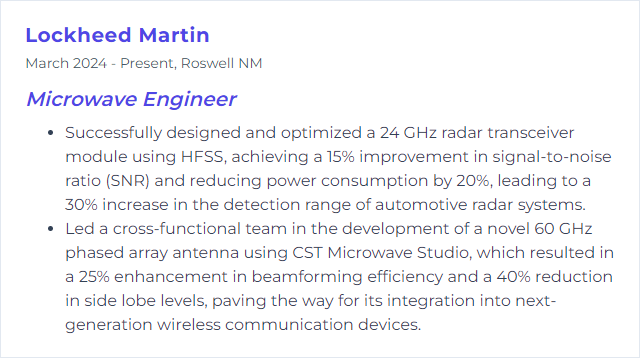
3. Antenna Theory
Antenna Theory is the study of the principles and concepts behind the design, operation, and performance of antennas. It encompasses understanding how electromagnetic waves are radiated, received, and how antennas can be optimized for specific frequencies and applications, such as in microwave engineering for efficient signal transmission and reception at microwave frequencies.
Why It's Important
Antenna Theory is essential for a Microwave Engineer because it provides the fundamental principles for designing, analyzing, and optimizing antennas, which are crucial for efficient microwave communication systems, radar, satellite, and RF applications, ensuring maximum signal transmission and reception with minimal loss or interference.
How to Improve Antenna Theory Skills
Improving your understanding of Antenna Theory as a Microwave Engineer involves a combination of theoretical study, practical experimentation, and continuous learning. Here’s a concise guide:
Strengthen Foundations: Begin with solidifying your grasp on electromagnetic theory and microwave engineering principles. The book "Foundations for Microwave Engineering" by Robert E. Collin is an excellent resource.
Dive into Antenna Theory: Delve into "Antenna Theory: Analysis and Design" by Constantine A. Balanis, a comprehensive guide that balances theoretical concepts with practical applications.
Simulation Tools: Familiarize yourself with antenna simulation software like ANSYS HFSS and CST Studio Suite. These tools help in designing, analyzing, and optimizing antennas.
Hands-On Projects: Apply your knowledge by building and testing antennas. Start with simple designs like dipoles or Yagi-Uda antennas. Document your process and results to understand the practical aspects and limitations.
Advanced Topics: Explore advanced topics and emerging trends in antenna design, such as MIMO systems, metamaterials, and antenna miniaturization. The IEEE Xplore Digital Library is a valuable resource for research papers and articles.
Professional Development: Join professional bodies like the IEEE Antennas and Propagation Society for access to the latest research, networking opportunities, and professional development resources.
Workshops and Conferences: Attend workshops, webinars, and conferences to stay updated on the latest trends, tools, and technologies in antenna theory and microwave engineering.
Peer Collaboration: Engage with the community through forums like Microwave Journal and RF and Microwave Community on Reddit, to share knowledge, discuss challenges, and explore innovative solutions.
By integrating these strategies, you can significantly improve your expertise in Antenna Theory and adapt to the evolving landscape of microwave engineering.
How to Display Antenna Theory Skills on Your Resume
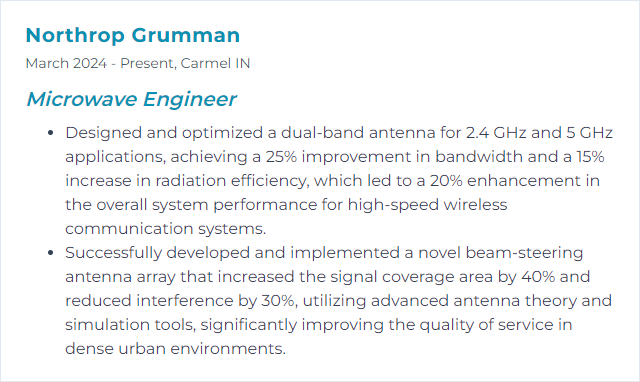
4. Network Analysis
Network Analysis, in the context of a Microwave Engineer, refers to the process of measuring, characterizing, and optimizing the performance of microwave components and systems. It involves the use of network analyzers to assess parameters such as scattering (S-parameters), impedance, and reflection, to ensure signal integrity and system efficiency in microwave frequencies.
Why It's Important
Network Analysis is crucial for a Microwave Engineer as it enables accurate measurement and characterization of microwave components and systems, ensuring optimal performance, signal integrity, and compliance with design specifications.
How to Improve Network Analysis Skills
To improve Network Analysis, a Microwave Engineer should focus on the following key areas:
Enhance Calibration Techniques: Regularly update and apply advanced calibration methods to ensure accurate measurements. Explore Maury Microwave's calibration solutions.
Use High-Quality Cables and Adapters: Invest in low-loss, phase-stable cables and high-quality adapters for minimal measurement errors. Check out Pasternack's high-performance cables.
Update Software Tools: Utilize the latest network analysis software for improved data analysis and visualization. Keysight's Network Analyzer Software offers comprehensive tools.
Understand the DUT's Characteristics: Deep knowledge of the Device Under Test (DUT) allows for better setup and analysis. IEEE Xplore provides resources for deeper understanding, such as IEEE Xplore Digital Library.
Continuous Learning: Stay updated with the latest trends and technologies in microwave engineering through courses and webinars, like those offered by IEEE Microwave Theory and Techniques Society.
By focusing on these areas, a Microwave Engineer can significantly improve the accuracy and reliability of network analysis.
How to Display Network Analysis Skills on Your Resume
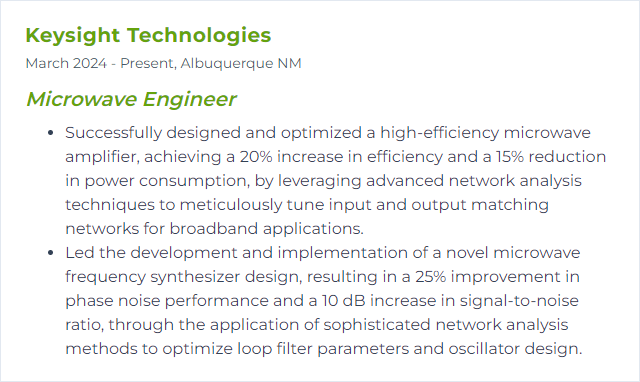
5. Signal Integrity
Signal integrity refers to the quality and reliability of an electrical signal as it travels through a circuit, ensuring it remains undistorted and free from unwanted noise or interference, particularly critical in high-frequency microwave systems for accurate data transmission and communication.
Why It's Important
Signal Integrity is crucial for a Microwave Engineer to ensure that high-frequency signals are transmitted with minimal distortion and loss, guaranteeing reliable communication and system performance over microwave frequencies.
How to Improve Signal Integrity Skills
Improving Signal Integrity involves several key strategies, particularly crucial in the field of microwave engineering. Here are concise pointers for enhancing Signal Integrity:
Use Proper Grounding: Ensure that your design incorporates a solid and continuous ground plane to minimize noise and interference. Grounding Techniques.
Impedance Matching: Match the impedance of your transmission lines with the load to minimize reflections. This is critical at microwave frequencies. Impedance Matching Network.
Minimize Crosstalk: Space parallel traces appropriately and use ground planes or shielding between signals when possible. Crosstalk Reduction Techniques.
Use High-Quality Components: Components with better performance characteristics can significantly reduce signal degradation. Microwave Component Selection.
Optimize PCB Layout: Keep high-speed traces short and direct, and consider the use of differential signaling where applicable. PCB Design for SI.
Employ Proper Filtering: Use filters to eliminate unwanted frequencies and reduce noise. RF Filtering Techniques.
These strategies, tailored for microwave engineering, help maintain signal integrity across complex systems.
How to Display Signal Integrity Skills on Your Resume
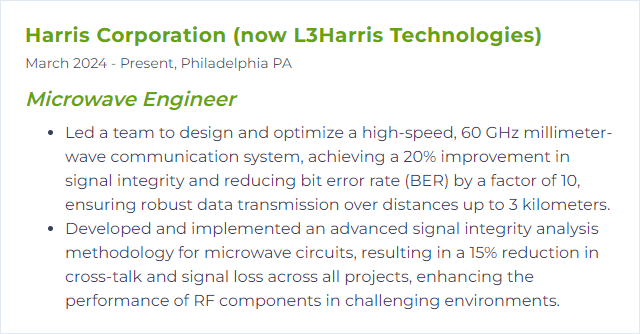
6. Electromagnetic Compatibility
Electromagnetic Compatibility (EMC) is the ability of electrical equipment and systems to function satisfactorily in their electromagnetic environment without introducing intolerable electromagnetic disturbances to other equipment in that environment. For a microwave engineer, EMC involves ensuring that microwave devices, such as antennas and transceivers, operate as intended without causing or experiencing interference from electromagnetic sources.
Why It's Important
Electromagnetic Compatibility (EMC) is crucial for a Microwave Engineer because it ensures that electronic devices operate without interference from or to other nearby devices, maintaining system integrity and performance across various environments.
How to Improve Electromagnetic Compatibility Skills
Improving Electromagnetic Compatibility (EMC) involves several strategies aimed at ensuring electronic devices operate without interfering with each other's functioning. For a Microwave Engineer, the focus should be on design optimization, proper shielding, filtering, and grounding techniques. Here's a concise guide:
Design Optimization: Minimize electromagnetic interference (EMI) at the source. Utilize circuit design techniques that reduce emissions and susceptibility, such as differential signaling and careful layout planning. More on High-Speed Digital Design for EMI Control.
Shielding: Implement electromagnetic shields around critical components or circuits to prevent EMI from entering or exiting. Materials like metals or conductive coatings are effective. Learn about EMI Shielding Techniques.
Filtering: Use filters to block unwanted frequencies from entering or exiting your device. Low-pass filters are commonly used to suppress high-frequency noise. Insight on EMI Filtering Techniques.
Grounding: Implement effective grounding strategies to provide a path for noise to return to the ground, minimizing its impact on the device's performance. Grounding techniques vary depending on the application and require careful consideration. Overview on Grounding for EMC.
Compliance Testing: Finally, rigorous testing against EMC standards ensures your design meets required guidelines and minimizes the risk of interference. Testing methodologies and standards can be found at IEC - Electromagnetic Compatibility.
By incorporating these strategies into the design and development phases, a Microwave Engineer can significantly improve the EMC of electronic devices, ensuring they meet regulatory standards and perform reliably in their intended environments.
How to Display Electromagnetic Compatibility Skills on Your Resume
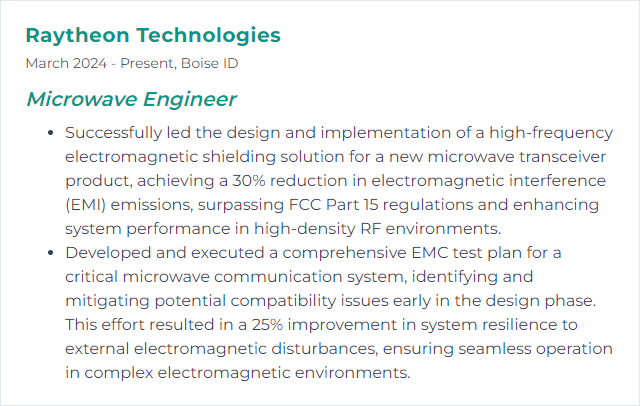
7. Spectrum Analysis
Spectrum analysis is the process of examining the magnitude of signal components versus frequency within a microwave system to identify, measure, and evaluate the frequency content and characteristics of signal emissions across a defined bandwidth.
Why It's Important
Spectrum analysis is crucial for a Microwave Engineer as it enables the identification and characterization of signal frequencies, bandwidths, and power levels within microwave systems, ensuring optimal performance, interference avoidance, and regulatory compliance.
How to Improve Spectrum Analysis Skills
To improve spectrum analysis as a Microwave Engineer, focus on the following short and concise strategies:
Enhance Resolution Bandwidth: Optimize the resolution bandwidth (RBW) settings to balance between measurement speed and the ability to resolve closely spaced signals. Lower RBW for better resolution, higher RBW for faster sweeps. More on RBW.
Utilize High-Quality Cables and Connectors: Minimize signal loss and VSWR (Voltage Standing Wave Ratio) by using high-quality, well-maintained cables and connectors. Cable and Connector Care.
Apply Appropriate Windowing: Use window functions (e.g., Hamming, Blackman) to reduce spectral leakage when analyzing non-continuous signals. This is crucial for accurate spectral analysis of transient or modulated signals. Window Functions Overview.
Leverage Averaging Techniques: Implement averaging techniques (time-domain or frequency-domain averaging) to reduce random noise in the spectrum, enhancing signal visibility. Understanding Averaging.
Optimize Detector Settings: Select the appropriate detector mode (e.g., peak, average, sample) based on the signal characteristics and the analysis goal. Peak for capturing transients, average for noise reduction. Detector Modes Explained.
Use Pre-amplification: When dealing with low-level signals, consider using a pre-amplifier to boost signal levels before analysis, improving the signal-to-noise ratio (SNR). Pre-amplification Benefits.
Software Tools and Updates: Utilize the latest spectrum analysis software and firmware updates for your equipment, taking advantage of improved algorithms and features for signal analysis. Keeping Firmware Updated.
Implementing these strategies will lead to more accurate and effective spectrum analysis in microwave engineering applications.
How to Display Spectrum Analysis Skills on Your Resume
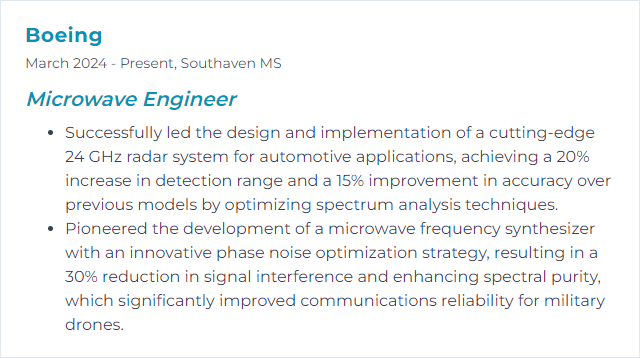
8. Microwave Photonics
Microwave Photonics is an interdisciplinary field that combines microwave engineering and photonics to explore and exploit the interaction between microwave signals and optical signals. It focuses on the generation, transmission, processing, and measurement of microwave signals using photonic devices and techniques, aiming to leverage the wide bandwidth and low-loss transmission offered by optical systems for applications in areas such as telecommunications, radar, and sensing.
Why It's Important
Microwave Photonics is crucial for a Microwave Engineer as it enables the integration of microwave and optical systems, offering advantages such as wide bandwidth, low loss, and immunity to electromagnetic interference. This integration enhances the performance and functionality of communication and radar systems, facilitating high-speed, high-capacity signal processing and transmission over long distances without significant degradation.
How to Improve Microwave Photonics Skills
To improve Microwave Photonics as a Microwave Engineer, focus on the following strategies:
Enhance Bandwidth and Frequency Agility: Work on expanding the operational bandwidth and improving frequency agility through advanced modulator designs. Consider Integrated Photonics approaches for compactness and efficiency. Integrated Microwave Photonics provides insights into combining microwave engineering with photonic integration.
Improve Noise Performance: Focus on lowering phase noise in microwave photonic systems. Techniques include optimizing oscillator design and incorporating high-quality factor (Q-factor) optical resonators. Phase Noise in Microwave Photonics offers strategies for reducing noise.
Increase System Efficiency: Enhance the efficiency of microwave photonic systems by improving the conversion efficiency between the optical and microwave domains. This can be achieved through better optoelectronic devices. Efficient Microwave to Optical Photon Conversion discusses advancements in this area.
Leverage Digital Signal Processing (DSP): Implement advanced DSP techniques to compensate for impairments and enhance the performance of microwave photonic systems. DSP in Microwave Photonics highlights the role of DSP in improving system performance.
Explore Novel Materials and Structures: Investigate the use of emerging materials (e.g., graphene, 2D materials) and photonic crystal structures to develop innovative microwave photonic devices with superior properties. Graphene for Microwave Photonics is a valuable resource.
By focusing on these areas, you can contribute to the advancement of microwave photonics, leading to systems with broader bandwidth, higher efficiency, lower noise, and enhanced functionalities.
How to Display Microwave Photonics Skills on Your Resume
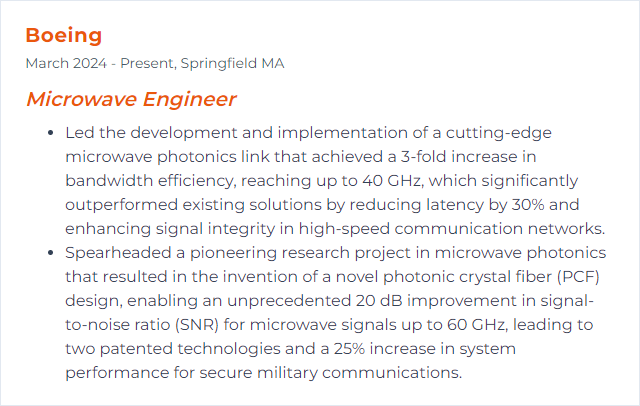
9. MMIC Design
MMIC Design refers to the process of creating Monolithic Microwave Integrated Circuits, which are specialized circuits designed to operate at microwave frequencies (typically 300 MHz to 300 GHz). This involves integrating active and passive components (such as transistors, resistors, capacitors, and inductors) on a single semiconductor substrate, using techniques tailored to achieve high performance in terms of gain, noise, and bandwidth for applications in radar, communications, and sensing.
Why It's Important
MMIC (Monolithic Microwave Integrated Circuit) design is crucial for Microwave Engineers as it enables the integration of multiple microwave functions into a single chip, significantly improving performance, reducing size and cost, and enhancing reliability for high-frequency applications.
How to Improve MMIC Design Skills
Improving Monolithic Microwave Integrated Circuit (MMIC) design involves a multifaceted approach focusing on optimization of the design process, incorporation of advanced simulation tools, and adherence to best practices in microwave engineering. Here are concise strategies:
Utilize Advanced Simulation Tools: Employ state-of-the-art electromagnetic simulation software like ANSYS HFSS for accurate modeling of electromagnetic fields and circuit behaviors, ensuring design robustness.
Optimize Component Layout: Minimize parasitic effects by optimizing component layout. Tools like Cadence provide layout optimization features that can help in reducing signal loss and crosstalk.
Material Selection: Choose substrate materials with appropriate dielectric properties for your frequency range. This guide from Rogers Corporation offers insights into selecting materials for high-frequency applications.
Thermal Management: Implement designs that enhance thermal dissipation. Techniques such as using via holes for heat transfer can be crucial. Qorvo’s design considerations offer practical insights into thermal management in MMIC designs.
Incorporate Design for Manufacturing (DFM): Early in the design process, consider manufacturing limitations and costs. Keysight’s DFM principles can guide you in making designs more manufacturable.
Iterative Design and Testing: Adopt an iterative approach to design, testing each version thoroughly. This practice helps in identifying and rectifying design flaws early.
Continuous Learning and Training: Stay updated with the latest technologies and design strategies by engaging in continuous learning. Online platforms like IEEE Xplore provide access to the latest research and developments in MMIC design.
By integrating these strategies, microwave engineers can significantly improve the performance, efficiency, and manufacturability of MMIC designs.
How to Display MMIC Design Skills on Your Resume
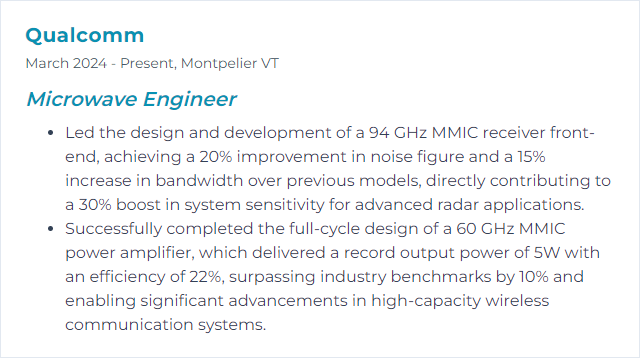
10. Radar Systems
Radar Systems are electronic systems that use radio waves to detect, locate, and measure the velocity of objects. They operate by transmitting microwave signals, which bounce off targets and return echoes that are analyzed to gather information about the target's distance, speed, and direction.
Why It's Important
Radar systems are crucial for microwave engineers because they enable precise detection, ranging, and imaging of objects through electromagnetic wave reflection, essential for applications in navigation, surveillance, weather monitoring, and collision avoidance.
How to Improve Radar Systems Skills
Improving radar systems involves enhancing their resolution, sensitivity, and reliability through various technical strategies. Here are concise suggestions tailored for a Microwave Engineer:
Increase Bandwidth: Wider bandwidths improve the range resolution by allowing the radar system to distinguish between closely spaced targets. Enhancing Bandwidth can be achieved through advanced pulse compression techniques and utilizing broader frequency ranges.
Adopt Adaptive Signal Processing: Implement adaptive beamforming and clutter rejection techniques to improve the signal-to-noise ratio (SNR) and target detection capabilities. Adaptive Signal Processing Techniques can dynamically optimize the radar's performance in real-time.
Utilize MIMO Radar Technology: Multiple-input multiple-output (MIMO) radar systems use multiple transmitters and receivers to enhance spatial resolution and target detection. MIMO Radar provides significant improvements in angle estimation and target localization.
Incorporate Advanced Materials: Employ advanced materials, such as metamaterials, to design antennas and radomes that can reduce size, weight, and improve the efficiency of radar systems. Metamaterials in Radar offer novel ways to manipulate electromagnetic waves for better performance.
Implement Cognitive Radar: Cognitive radar systems adaptively learn from the environment to optimize their detection strategies, improving reliability and efficiency. Cognitive Radar leverages AI and machine learning algorithms for dynamic performance enhancement.
Enhance Thermal Management: Effective thermal management is crucial for maintaining the performance and longevity of radar systems, especially those utilizing high-power transmitters. Thermal Management Techniques can include advanced cooling systems and heat sink designs.
Foster Interoperability and Standardization: Ensuring that radar systems can operate effectively in a network-centric environment enhances their overall utility. Interoperability Standards facilitate seamless integration with other systems and platforms.
By focusing on these areas, Microwave Engineers can significantly improve the performance and capabilities of radar systems, addressing the evolving demands of modern applications.
How to Display Radar Systems Skills on Your Resume
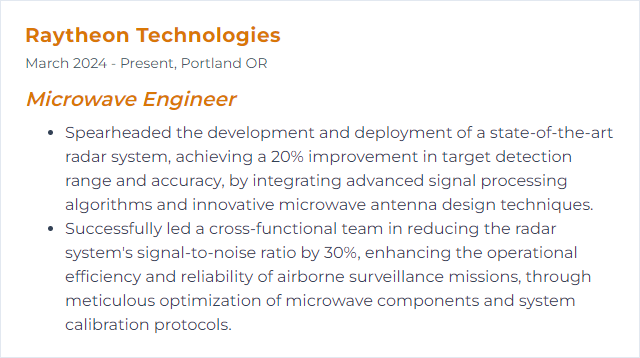
11. Waveguide Technology
Waveguide technology refers to the use of hollow metallic or dielectric conduits to guide electromagnetic waves, particularly microwaves, from one point to another with minimal loss. These structures support specific modes of propagation, allowing for efficient energy transfer in microwave systems and devices, including radars, satellite communications, and microwave transmission lines.
Why It's Important
Waveguide technology is crucial for microwave engineers because it efficiently guides high-frequency electromagnetic waves with minimal loss, enabling precise control and distribution of microwave signals in radar, communication systems, and microwave transmission lines.
How to Improve Waveguide Technology Skills
Improving waveguide technology, particularly for microwave engineers, involves enhancing efficiency, minimizing losses, and expanding bandwidth. Here are concise strategies:
Material Optimization: Use low-loss materials like silver or aluminum coatings for internal surfaces to reduce ohmic losses. ResearchGate.
Geometry Adjustments: Optimize the shape and dimensions of the waveguide to support the desired mode efficiently while suppressing unwanted modes. Rectangular and circular waveguides are most common, but elliptical or other shapes can offer unique advantages. IEEE Xplore.
Surface Roughness Reduction: Improve the manufacturing process to minimize surface roughness, which can significantly reduce scattering losses, especially at higher frequencies. ScienceDirect.
Dielectric Loading: Inserting low-loss dielectric materials can effectively lower the cut-off frequency, enabling the waveguide to operate efficiently over a broader frequency range. IEEE Xplore.
Advanced Fabrication Techniques: Employ modern fabrication technologies like 3D printing to create complex waveguide structures that are otherwise difficult to manufacture, offering novel ways to control wave propagation. MDPI.
Hybrid Integration: Combine waveguides with other components like filters and antennas directly into the waveguide structure to reduce insertion losses and improve overall system performance. Frontiers.
By focusing on these areas, microwave engineers can significantly enhance the performance and application range of waveguide technology.
How to Display Waveguide Technology Skills on Your Resume
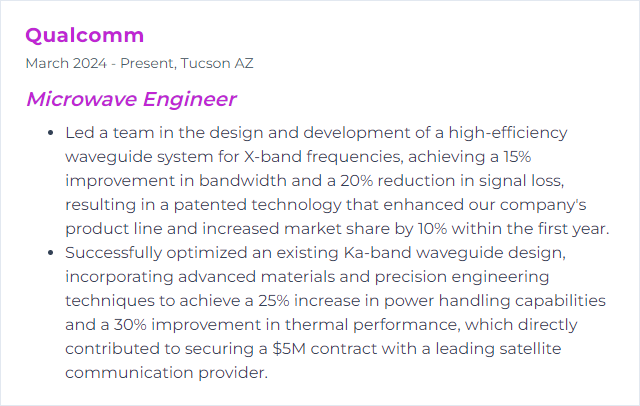
12. S-Parameters Analysis
S-Parameters Analysis is a method used in microwave engineering to describe the electrical behavior of linear RF and microwave devices, encompassing reflection and transmission characteristics across ports. This analysis involves measuring scattering parameters (S-parameters), which are complex numbers indicating how much of an incident signal is reflected from or transmitted through the device, facilitating the design and characterization of components like amplifiers and antennas.
Why It's Important
S-parameters (Scattering parameters) analysis is crucial for microwave engineers because it provides a standardized way to describe how RF (Radio Frequency) networks behave in terms of signal reflection and transmission, enabling the efficient design, analysis, and optimization of microwave circuits and systems for performance, stability, and matching.
How to Improve S-Parameters Analysis Skills
Improving S-Parameters (Scattering Parameters) Analysis involves enhancing measurement accuracy, simulation models, and interpretation of results for microwave circuits. Here’s a concise guide:
Calibration and Measurement Accuracy: Use proper calibration techniques to minimize errors in measurements. Vector Network Analyzers (VNAs) should be calibrated using standards for better accuracy. Consider employing TRL (Thru-Reflect-Line) calibration for non-coaxial measurements. Keysight’s Calibration Guide provides insights into effective VNA calibration methods.
Simulation and Modeling: Improve simulation accuracy by using high-fidelity models and incorporating substrate effects, parasitics, and 3D electromagnetic simulation when necessary. Tools like ANSYS HFSS offer advanced modeling capabilities.
De-embedding Techniques: To focus on the device under test (DUT), use de-embedding to remove the effects of fixtures and cables. This enhances the relevance of S-Parameters to the actual device performance. Microwave Journal’s De-embedding Tutorial explains this process in detail.
Broadband and Multiport Measurements: For devices operating over a wide frequency range or having multiple ports, ensure that your analysis considers the entire operating bandwidth and interactions between all ports. Tools like NI AWR help in predicting critical S-Parameters accurately.
Temperature and Non-linear Effects: Include temperature variations and non-linear effects in your analysis for components like amplifiers or mixers. Software such as Cadence AWR Microwave Office allows for non-linear simulation and analysis.
Documentation and Reporting: Clearly document measurement conditions, assumptions, and any corrections applied. This ensures repeatability and reliability of the S-Parameters analysis. Use standardized reporting formats where possible.
By focusing on accurate calibration, advanced simulation techniques, and comprehensive analysis, microwave engineers can significantly improve the quality and reliability of S-Parameters analysis.
How to Display S-Parameters Analysis Skills on Your Resume
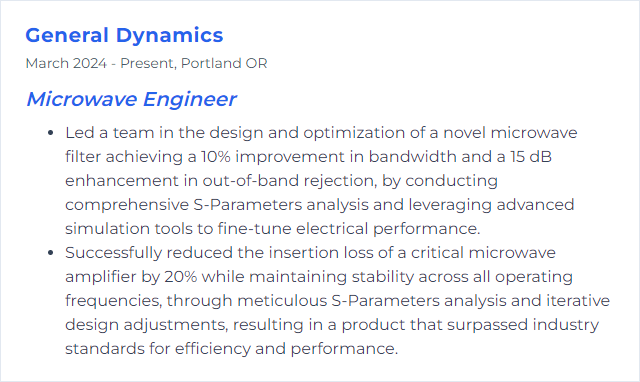